The
all-or-none law states that:
...when stimulated, nerve fibers invariably produce a response of a single, maximal amplitude.
In the late 19
th Century, the great American physiologist Henry Pickering Bowditch was studying the contractions of heart muscles in response to electrical stimulation. It had long been assumed that cardiac muscles can contract to differing degrees dependent upon the stimulation—the more stimulation, the greater the contraction. But, to his surprise, Bowditch discovered a very different mechanism—if the electrical stimulus was adequate, the heart contracted with
maximum power; if the stimulus was inadequate, the cardiac muscles
didn’t contract at all. It didn’t matter how much voltage he pumped into the heart tissue, whether a huge dose or tiny trickle; the results were the same—the heart either contracted fully or not at all. It was all, or nothing.
A few short decades later, the British neuroscientist Keith Lucas applied similar experiments to individual muscle cells. He quickly realized that the individual fibers, when stimulated, behave in exactly the same way as little hearts—they either contract maximally, or not at all. This phenomenon was swiftly found to be a universal principle of muscle activity, and later named the
all-or-none law. It is considered one of the fundamental laws of myology.
On the face of it, this strange law does not seem to have very much to do with bodybuilding or strength training. But, in reality, it forms the
cornerstone for all resistance training. In order to comprehend resistance training at even a basic level, we must understand this law inside and out.
The binary nature of muscle cells
Perhaps a simpler way to explain the all-or-none law for our purposes is to say that muscle cells are binary. Like a simple light switch, they possess two states: either on, or off. When muscle cells are stimulated—i.e., "on"—they contract as hard as possible; when they are not stimulated—i.e., "off" they are completely relaxed.
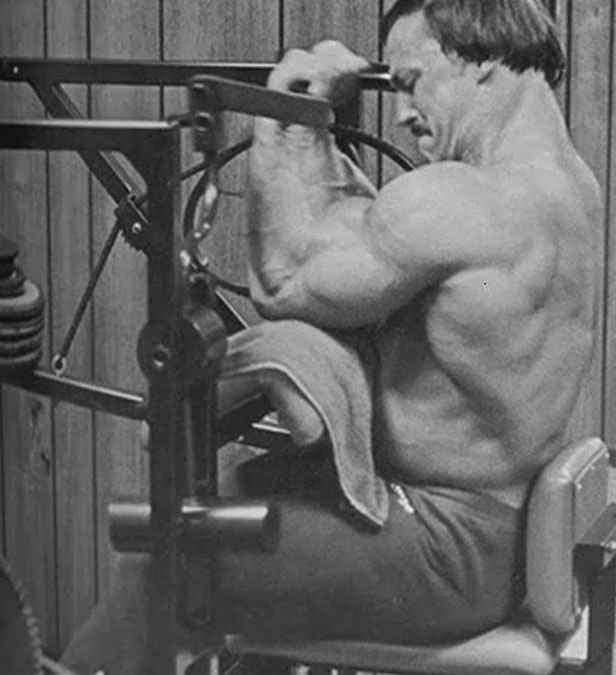
At first glance, this notion might seem counterintuitive. In everyday experience, our muscles do not appear to have just two levels of stimulation—maximum and off. Rather than being binary in nature, like a toggle light switch, they seem to work more like dimmer bulbs—they can be turned "on" at any level of tension, from extremely light and barely noticeable, all the way up to maximum force, as well as every conceivable level in between. How is this possible, if the basic component of our muscles—the cells—are binary?
Our muscles are composed of many, many fibers (muscle cells and muscle fibers are essentially synonymous. A motor unit is a group of muscle fibers innervated by a single motor nerve, or neuron). How hard a muscle contracts—how much force or tension it generates—depends upon how many of those fibers contract. It has nothing to do with how hard the individual fibers contract; when a muscle fiber contracts, it contracts at maximum strength, or not at all.
Force generation = fiber recruitment
Every human being possesses varying total numbers, types, and strengths of muscle fibers; but as an inviolable rule, we all generate more force (i.e., muscular tension) by recruiting a greater portion of those fibers we possess.
The biceps brachii, for example—the classic "bodybuilder muscle" of the upper arm—contains, on average, approximately 300,000 muscle fibers. If you twitch your biceps as gently as possible, perhaps only a few hundred of those fibers are necessary to perform this action. Then let’s say you curl a 10 lbs dumbbell—now thirty thousand fibers are at work. You perform a pull-up, and a hundred thousand fibers are recruited to execute the drill. Then imagine you are holding up a burning vehicle to allow a child to escape—straining to the utmost limit—now over a quarter of a million of those individual biceps fibers are firing together.
The more fibers are recruited, the more force the muscles generate.
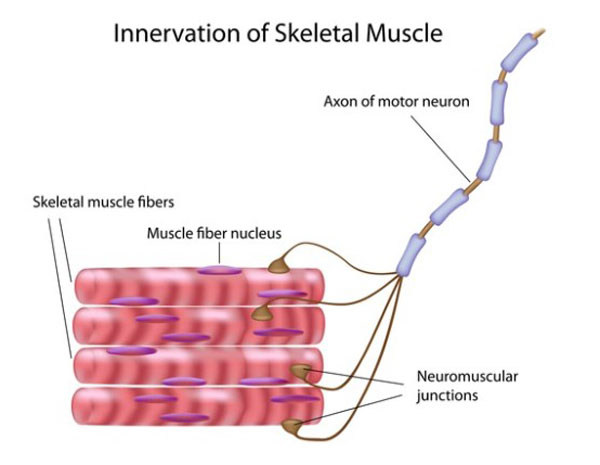
Interestingly, the number of muscle fibers we possess remains relatively stable throughout our adult life. We do not become stronger by adding extra muscle fibers (a process which can occur in other organs, called hyperplasia). Rather, we become stronger primarily because our brains learn to recruit more of the cells we already possess. This learning process is described by a physiological principle known as Hebbes’ law, which will be covering in-depth in a later article in this series.
Muscles deal in force—not movement
A simple—if shocking—corollary of the all-or-none theory is that
muscles neither register nor regulate movement.
All muscles do is produce varying degrees of force, depending upon the proportion of fibers recruited. Whether that amount of force results in
motion entirely depends upon the
force-velocity relationship inherent in any given case. If you are raising your arm to wave to a friend, only a small number of fibers will be required for your arm to shoot upwards. If you are lifting a heavy dumbbell, many thousands of fibers might need to be recruited for your arm to move upwards, even slowly. If you are shackled to a wall, virtually all of your muscle cells may be firing, with zero net movement resulting.
Muscles deal only in force production—which, remember, is determined by the proportion of fibers recruited. Movement is regulated by the
brain, via the nervous system, not the
muscles.
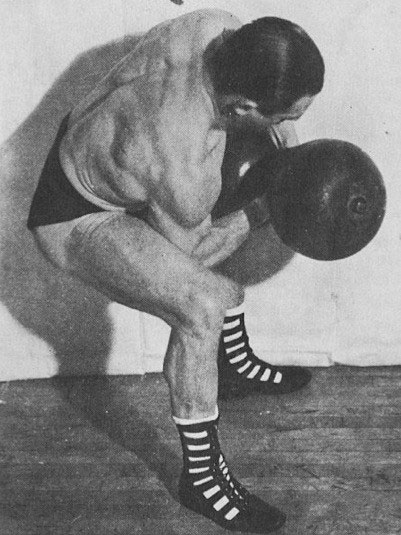
This concept will immediately feel alien to anyone immersed in a culture of bodybuilding or strength training. We are taught that we train our muscles by
moving—specifically, moving weights up and down. However, the message of the science on this matter is crystal clear: the muscles are only trained by
producing force—recruiting the maximal number of muscle cells. Movement not only has nothing to do with this, it is actually counter-productive. If you have absorbed the
previous article in this series—regarding the force-velocity relationship—you will understand that maximal forces
can only be produced by the muscles when stationary. Muscle movement
can only occur when resistance is sub-maximal. This is an absolute law of biophysics.
Naturally, if you wish to become better at
movement, you must train
movements. If you wish to be the best dancer you must dance; if you wish to be the best Olympic weightlifter, you must practice the movements which comprise the sport. The same is true for powerlifting, etc. However, if you want to train your muscular system to maximal strength and/or size, movement is not desirable. What is required is a method of training which recruits the maximal number of fibers possible, with zero movement involved. Ideally, this method will permit us to measure the forces involved with digital accuracy, to establish goals and track progress.
There is, of course, a method of training the muscles in this manner; this method is
Isochain isometrics, and it is the superior approach to strength training and bodybuilding. This philosophy can be summarized very simply:
Those five points comprise the essence of scientific isometric strength training in a nutshell. If they are followed correctly, the result is the greatest amplification of absolute strength humanly possible, within the most compressed time period. This has been proven in studies, over and over.
These points do not completely address hypertrophy—muscle growth—however. In order to understand the theory behind muscle growth, we must examine a further law of physiology: Henneman’s size principle.
We will cover this law in the next article in the series.